Up: Evaluation of Devasthal site
Subsections
As a follow up on the site survey reported above, precipitable
water vapour, meteorological and astronomical seeing measurements were
carried at Devasthal sites. Primary emphasis was placed on the evaluation
of ground level seeing at the two sites mentioned above.
Simultaneous measurements of atmospheric parameters such as air temperature,
soil temperature, solar radiation, relative humidity and wind speed
and direction as well as microthermal measurements were performed at Site 1.
These parameters are of major
importance in the interpretation of the occurrence of optical turbulence.
The precipitable water vapour content in the atmosphere was measured with the
instrument designed and built by Prof. Westphal and kindly loaned to UPSO. The observations were taken
between January 1989 to June 1989 at Devasthal.
They indicate 1-2 mm, 2-3 mm and
> 3 mm of precipitable water vapour for 21%, 43% and 36% of the
time respectively.
This section describes the DIMM instruments which were built in UPSO
laboratories
and used for seeing measurements
along with the procedure used for data processing.
Differential image motion technique has been used as early as 1960 to
provide quantitative seeing estimates.
Such work carried out in recent years
include those by the European
Southern Observatory (Sarazin & Roddier 1990), the National Optical
Astronomical Observatories (Forbes et al. 1988), the ESO site testing
measurements for the Very Large Telescope (Pedersen et al. 1988),
Observatorio del Roque de los
Muchachos (ORM ) at La Palma (Vernin & Muñoz-Tuñón 1995) etc.
for the evaluation of
potential observing sites.
The principles in detail of DIMM instrument can be found
in Sarazin & Roddier (1990).
A 52 cm telescope with an equatorial fork mounting having a plate scale of
31
/mm at the f/13 folded Cassegrain focus is used at Site 1,
whereas at Site 2, a 38 cm telescope with single pier mounting having a plate scale of
36
/mm
at the f/15 Cassegrain focus is used.
The
front portion of the 52 cm reflector tube is covered by a mask which has two
circular holes, each of 6 cm diameter and separated by 40 cm. Similarly, the
38 cm reflector is covered by a mask having two circular holes each of
5 cm in diameter and separated by 24 cm.
One of the holes contains a prism which deviates the incoming parallel light
of a star by about 30
in the direction joining the line of the centers of the two
holes, so that two images of the same star are formed on the CCD detector. The
telescopes are equipped with a PC which controls the Santa Barbara Instrument
Group (SBIG) ST4 autoguiding CCD camera and thus accumulates image motion
data and analyse them online to provide seeing measurements in two mutually
perpendicular directions. One pixel of the CCD corresponds to 0
42
0
49 at 52 cm telescope and 0
50
0
58 at 38 cm telescope. Our setup closely follows that of
Wood et al. (1995).
The details of the instruments used in the seeing campaign and their
characteristics are given in Table 2.
In DIMM, a series of exposures each spanning a period of
10 ms are taken and hundred such exposures are used to derive one
estimate of the standard deviation of the relative separation of the images,
and
parallel
and perpendicular to the line joining the centres of the two apertures.
Using Eqs. (3) and (4) one can then find independent
estimates of r0. These independent values of r0 are then
converted into seeing estimates using Eq. (1) and a wavelength of 5000 Å.
All the above processes are done online and the data are stored in the PC
for further analysis.
The data obtained using DIMM were then corrected for airmass (X)
using the
relation
 |
(5) |
The use of this correction is necessary as it allows comparisons to be made
with observations obtained at different time in various directions.
The uncertainty in the determination of centroid of an image
due to detector noise introduces an error in the seeing
measurements. Since this instrumental noise is not correlated with the
true nature of the atmosphere, this has to be subtracted out before an
estimation of the seeing is made (cf. Sarazin & Roddier 1990). The
estimation of the instrumental noise was carried out experimentally
in the optics lab of UPSO.
The intensity levels
of the images were kept similar to those that we maintain in performing
seeing measurements.
The uncertainty measured in the separation of the
images of two pin holes on the CCD detector gives a value of 0.09 pixel.
This system noise when taken into account improves our
seeing reported here by 0
01 only.
The statistical errors of the seeing measurements were calculated using the
formalism given by Frieden (1983). It has been pointed out by Sarazin
& Roddier (1990) that the statistical properties of the atmosphere does not
change for about a minute. Under typical seeing measurements at Devasthal
we are able to process about 50 images in a minute. The number of images
obtained in a minute is limited by the exposure time as well as the readout
time of the CCD. For our measurements the statistical error turns out to be
12%, and the error for each individaul measurement is about 9%.
![\begin{figure}\includegraphics[width=8cm]{ds8858f4.eps}\end{figure}](/articles/aas/full/2000/11/ds8858/Timg32.gif) |
Figure 4:
Seeing at Devasthal Site 2 plotted against UT.
Other details are the same as in Fig. 3 |
Table 3:
Results of seeing measurements at Devasthal
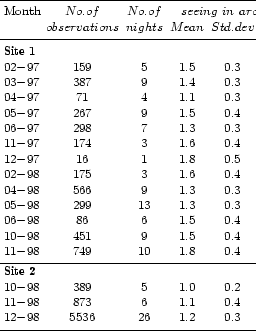 |
![\begin{figure}\includegraphics[width=8cm]{ds8858f5.eps}\end{figure}](/articles/aas/full/2000/11/ds8858/Timg34.gif) |
Figure 5:
Cumulative distribution and histogram of seeing measured
during the indicated month at
Devasthal Site 1. The solid and dotted lines
are for the year 1997 and 1998 respectively |
![\begin{figure}\includegraphics[width=8cm]{ds8858f6.eps}\end{figure}](/articles/aas/full/2000/11/ds8858/Timg35.gif) |
Figure 6:
Cumulative distribution and histogram of seeing measured during the
indicated month at Devasthal Site 2 |
![\begin{figure}\includegraphics[width=8cm]{ds8858f2.eps}\end{figure}](/articles/aas/full/2000/11/ds8858/Timg29.gif) |
Figure 7:
Cumulative distribution and histogram of entire seeing
measurements obtained at UPSO and Devasthal sites |
![\begin{figure}\includegraphics[width=8.8cm]{ds8858f8.eps}\end{figure}](/articles/aas/full/2000/11/ds8858/Timg36.gif) |
Figure 8:
Histogram and cumulative distribution of the wind speed at
Devasthal Site 1 |
![\begin{figure}\includegraphics[width=5cm]{ds8858f9.eps}\end{figure}](/articles/aas/full/2000/11/ds8858/Timg37.gif) |
Figure 9:
Distribution of wind speed and direction
at Devasthal Site 1 |
![\begin{figure}\includegraphics[width=8.8cm]{ds8858f10.eps}\end{figure}](/articles/aas/full/2000/11/ds8858/Timg38.gif) |
Figure 10:
Monthly variation of average relative humidity at Devasthal Site 1.
The solid and dotted lines represent the relative humidity during
day and night time respectively |
![\begin{figure}\includegraphics[width=8.8cm]{ds8858f11.eps}\end{figure}](/articles/aas/full/2000/11/ds8858/Timg39.gif) |
Figure 11:
Histogram and cumulative distribution of relative
humidity at Devasthal Site 1.
The solid line is for the whole data set, whereas
the dotted line is for the data set excluding the monsoon months |
For ESO DIMM the seeing values obtained from
and
agree with each other within 12%
(see Sarazin & Roddier 1990).
An attempt has been
made by us to see the behaviour of longitudinal and
transverse seeing for the
observations at Devasthal sites.
The seeing measurements were carried out for 88 nights at Site 1 during
February 1997 and November 1998, and for 37 nights at Site 2
between October and December 1998. In Fig. 2 we
have plotted the longitudinal seeing against the transverse seeing for
both Devasthal sites. For comparison, we have drawn a line of unit slope
and zero intercept.
This indicates a fairly good agreement between the
two measurements considering the errors discussed above.
Amongst the
two independent measurements, we have
therefore considered only one, namely the longitudinal component as the
seeing of the site. There is however some difference between the values
obtained from the two components of image motion; wind speed and the finite
outer scale length will affect the correlation between the two apertures
and could lead to this difference (see Das et al. 1999).
Seeing measurements were carried out for 88 and 37 nights at Site 1 (during
February 1997 to November 1998) and Site 2 (between October and December 1998)
respectively.
We have plotted in Fig. 3
the nightly variation of seeing against UT
for Site 1 while the same for Site 2
has been plotted in Fig. 4.
The statistical parameters obtained from seeing measurements
for each month such as mean, median etc.
are given in
Table 3.
They indicate that the seeing is
relatively better during April, May and June as compared to other
months. This tells us about a possible seasonal dependence of seeing, with
summer months showing an average seeing better than other months.
The monthwise histogram and cumulative distribution of seeing observations
at Devasthal Site 1 and Site 2 are shown in Figs. 5 and 6
respectively.
A series of test observations with the 52 cm telescope, which was later
shifted to Devasthal Site 1 was carried out for 18 nights
at UPSO, during November and December, 1996.
Figure 7 shows the histogram and cumulative
distribution of seeing data obtained at UPSO
which indicates a median value of
1
6. For comparison, corresponding plots for Devasthal sites are
also shown in Fig. 7.
Table 4 gives the overall statistics of the seeing values for the entire
observing run at UPSO and Devasthal sites.
A Comparison of seeing measured quasi-simultaneously at both Devasthal
sites on 8 nights during October and November 1998, is presented in
Table 5. The differences in median seeing values range from 0
16
to 1
24, with better seeing values always at Site 2. Figure 7, Tables 3
and 4 also indicate that Site 2 has better seeing values than Site 1. It
is worth
mentioning here that at Site 2 in comparison to Site 1, for significantly
large fraction (
40%) of the observing time seeing is < 1
(see Table 4). Another factor which favours Site 2 is
that the seeing measurements were done at about 2 m above the ground
level whereas at
Site 1 it was carried at about 4 m above the ground. Studies by
Pant et al. (1999), Vernin & Muñoz-Tuñón (1994) and Avila et al. (1998) indicate appreciable degradation
in seeing due to turbulence introduced by the surrounding trees, local
topography and ground radiation. The seeing improves considerably
at height
10 m above the ground as discussed below.
The detection of the local source of seeing degradation which occur in
levels of the atmosphere very near the ground, within a few tenths of metres
above ground is of great importance for evaluating the seeing conditions of an
astronomical site. Microthermal fluctuations at Devasthal Site 1 were
therefore recorded using
pairs of microthermal sensors made from Nickel wire of 25
in diameter
separated by a distance of 1 m and mounted at three different levels on
a mast situated respectively at 6, 12 and 18 m above the
ground. A description of the experimental setup and the methods employed
in deducing the seeing estimates from the microthermal measurements can be
found in Pant & Sagar (1998) and Pant et al. (1999).
Observations of the surface
layer contribution to seeing were carried out during March to June 1998.
The results of these observations have
been reported by Pant et al. (1999) where they found that the major
contribution to seeing comes from the 6 - 12 m slab of the
atmosphere and sub-arcsec seeing of 0
65 and 0
5 can be
achieved by locating the telescope at a height of
13 m
and 18 m above the ground respectively. Similar conclusion about the
degradation of image due to surface layer turbulence is also given by
Vernin & Muñoz-Tuñón (1994). There is a plan to carry out similar
measurements at Devasthal Site 2 also.
![\begin{figure}\includegraphics[width=8.8cm]{ds8858f12.eps}\end{figure}](/articles/aas/full/2000/11/ds8858/Timg41.gif) |
Figure 12:
Monthly variation of average air temperature at
Devasthal Site 1. The solid and dotted lines represent the diurnal and
nocturnal air temperature respectively |
Measurements of
atmospheric parameters such as temperature, humidity, wind etc. simultaneous
with DIMM and microthermal observations are of
major importance in the interpretation of the atmospheric turbulence. To accomplish
this a meteorological Automatic Weather Station (AWS) is also established close to the
52 cm
telescope and the microthermal tower.
The instrument is from M/s Champbell Scientific Inc. from U.S.A. It
contains wind speed sensors, wind direction sensors, pyranometer for solar
radiation measurement, temperature sensors for sensing the air and soil
temperature, electronic tripping bucket rain gauge, relative humidity meter,
solar panel for charging a 12 volt battery and data logger.
All the above instruments are mounted at the appropriate places on a mast
fitted in a tripod. The tripod is kept upright and oriented, so one leg
points due south, and then plumb the mast by adjusting south and northeast
facing legs. The tripod including the mast is grounded with the help of a
ground rod.
The instruments and the data logger are connected to a 12 volt battery to
record the above mentioned meterological data automatically in a module.
The data are stored in the module at one hour interval through programming.
The
capacity of the module is to store data for about a month. After a month the
module is taken out of the data logger for processing and another module
is connected for further recording.
The meteorological data thus
obtained are useful to see if there is any effect of these parameters on
seeing.
The histogram and cumulative distribution of day and night wind speed at
Site 1 are shown
in Fig. 8. It is found that
both diurnal and nocturnal wind speed are less than 3 m/s for 85%
and 87% of the time respectively. The prevailing wind direction
is mostly NW and it seldom exceeds 10 m/s. This is in agreement with
our earlier observations(see Sect. 2.1.4).
The distribution of wind speed and direction is shown in
Fig. 9. The monthly statistics of average relative humidity
are shown in Fig. 10 for both day and night. Figure 11 shows
the histogram and cumulative distribution of relative humidity for the
whole observing
period.
Both day as well as night time measurements indicate that 55% of the time,
relative humidity
lies below 85%. However, this fraction increases to 70% if we
exclude monsoon months from July to September.
The monthly variation of nocturnal and diurnal average
air temperature is given in Fig. 12. The difference between
day and
night average temperature is less than 3
C for all the
months except March, April, May and June, where it
is somewhat higher but still less than 6
C.
![\begin{figure}\includegraphics[width=8.8cm]{ds8858f13.eps}\end{figure}](/articles/aas/full/2000/11/ds8858/Timg42.gif) |
Figure 13:
Average seeing of 30 min duration versus UT for the entire observing period at
Devasthal |
Table 5:
A comparison of median seeing values measured at both Devasthal
sites. Number of measured data points are given inside bracket
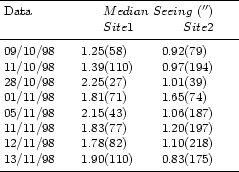 |
![\begin{figure}\includegraphics[width=8cm]{ds8858f14.eps}\end{figure}](/articles/aas/full/2000/11/ds8858/Timg44.gif) |
Figure 14:
Dependence of seeing on total solar radiation, wind direction,
wind speed, air temperature and relative humidity at Devasthal Site 1 |
From the point of view of astronomers, it is useful to
have a characterization of the temporal evolution of seeing, i.e., the
variation of seeing quality with time. The results of
all observing nights are averaged in 30 minutes bin and plotted against
UT in Fig. 13 for Site 1 and
Site 2. No significant trend is seen. This is in contrast
with the generally prevailing notion among astronomers that seeing is
poorer in the beginning of night and improves later in the night.
Our results for Devasthal is in agreement with what has been
found for the ORM site at La Palma by Muñoz-Tuñón
et al. (1997), where no general trend in the seeing evolution is noticed.
We have checked for possible relationships between the meteorology
and the image quality for Devasthal Site 1.
In Fig. 14, we have plotted the variation of seeing
with meteorological parameters namely total solar radiation, wind direction,
wind speed, air temperature and relative humidity.
We observe that relative
humidity does not seem to affect the seeing significantly.
Weak correlation is noticed between air temperature
and seeing, in the sense seeing improves at higher air temperature. A
marginal dependence of seeing on total solar radiation is noticed with
sunny days having better seeing. These are
consistent with results given in Table 3, where seeing is better in summer
months when air temperature is higher than that for rest of the year.
The interesting plot concerns the wind speed and direction following
the belief that these have an influence on the seeing.
Correlation of wind speed is expected but not for low wind speed as the
turbulence for wind speed less than 15 m/s is relatively unimportant
(Woolf 1982). Contrary to this, a weak correlation is noticed between seeing
and wind speed and direction.
The stability of seeing with time tells us about the quality of the site
To get a knowledge of the same we carefully looked into
individual nights having more than 6 hours of continuous seeing data.
This has been
done only for Site 2 as it is better
than Site 1. Among the
37 nights of observations at Devasthal Site 2 we have 25 nights which
satisfied this criterion.
The time during which seeing is less than
1
0 in a stretch for >4 hour,
2 to 4 hour and < 2 hour
are found to be 10%, 47% and 43% respectively.
Table 6:
Comparison of present seeing results with those of other sites
Site/Observatories |
Seeing (
) |
Instrument |
Source |
International sites |
|
|
|
|
|
|
|
Mt. Graham, Arizona, U.S.A. |
0.60 |
STTa |
Cromwell et al. (1998) |
Mt. Hopkins, Arizona, U.S.A. |
0.59 |
STT |
Cromwell et al. (1990) |
Las Campanas, Chile |
0.60 |
CMb |
Persson et al. (1990) |
La Silla, Chile |
0.87 |
DIMM |
Murtagh & Sarazin (1993) |
Paranal, Chile |
0.64 |
DIMM |
Murtagh & Sarazin (1993) |
Mauna Kea, Hawai, U.S.A. |
0.57 |
SCIDARc |
Roddier et al. (1990) |
La Palma, Spain |
0.64 |
DIMM |
Muñoz-Tuñón et al. (1997) |
SPM, B. C., Mexico |
0.61 |
STT, CM |
Echevarria et al. (1998) |
Freeling Heights, Australia |
1.20 |
DIMM |
Wood et al. (1995) |
Siding Spring, Australia |
1.20 |
DIMM |
Wood et al. (1995) |
|
|
|
|
Indian sites |
|
|
|
|
|
|
|
UPSO |
1.57 |
DIMM |
This work |
Devasthal Site 1 |
1.44 |
DIMM |
This work |
Devasthal Site 2 |
1.07 |
DIMM |
This work |
Leh |
1.07 |
DIMM |
Bhatt et al. (2000) |
IUCAA, Pune |
1.50 |
DIMM |
Das et al. (1999) |
a Site Testing Telescope.
b Carneige Monitor.
c SCIntillation Detection And Ranging.
Routine extinction measurements in standard passbands were
carried out using the 52 cm
reflector equipped with a solid state SSP3 photometer. The lowest value of
extinction was observed on 20 and 21 February, 1998. They are
0.40
0.01, 0.22
0.01, 0.12
0.01 and 0.06
0.01
in Johnson U, B, V and R bands respectively. A detailed paper on the
atmospheric extinction
measurements at Devasthal is published elsewhere
(cf. Mohan et al. 1999).
Up: Evaluation of Devasthal site
Copyright The European Southern Observatory (ESO)